Atomic Energy for Military Purposes (The Smyth Report)
CHAPTER XI. ELECTOMAGNETIC SEPARATION OF URANIUM ISOTOPES
INTRODUCTION
11.1. In Chapter IV we said that the possibility of large-scale separation of the uranium isotopes by electromagnetic means was suggested in the fall of 1941 by E. O. Lawrence of the University of California and H. D. Smyth of Princeton University. In Chapter IX we described the principles of one method of electromagnetic separation and listed the three limitations of that method: difficulty of producing ions, limited fraction of ions actually used, and space charge effects.
11.2. By the end of December 1941, when the reorganization of the whole uranium project was effected, Lawrence had already obtained some samples of separated isotopes of uranium and in the reorganization he was officially placed in charge of the preparation of further samples and the making of various associated physical measurements. However, just as the Metallurgical Laboratory very soon shifted its objective from the physics of the chain reaction to the large-scale production of plutonium, the objective of Lawrence's division immediately shifted to the effecting of large-scale separation of uranium isotopes by electromagnetic methods. This change was prompted by the success of the initial experiments at California and by the development at California and at Princeton of ideas on other possible methods. Of the many electromagnetic schemes suggested, three soon were recognized as being the most promising: the "calutron" mass separator, the magnetron-type separator later developed into the "ionic centrifuge," and the "isotron" method of "bunching" a beam of ions. The first two of these approaches were followed at California and the third at Princeton. After the first few months, by far the greatest effort was put on the calutron, but some work on the ionic centrifuge was continued at California during the summer of 1942 and was further continued by J. Slepian at the Westinghouse laboratories in Pittsburgh on a small scale through the winter of 1944-1945. Work on the isotron was continued at Princeton until February 1943, when most of the group was transferred to other work. Most of this chapter will be devoted to the calutron since that is the method that has resulted in large-scale production of U-235. A brief description will also be given of the thermal diffusion plant built to provide enriched feed material for the electromagnetic plant.
11.3. Security requirements make it impossible here - as for other parts of the project - to present many of the most interesting technical details. The importance of the development is considerably greater than is indicated by the mount of space which is given it here.
ELECTROMAGNETIC MASS SEPARATORS
PRELIMINARY WORK
11.4. A. O. Nier's mass spectrograph was set up primarily to measure relative abundances of isotopes, not to separate large samples. Using vapour from uranium bromide Nier had prepared several small samples of separated isotopes of uranium, but his rate of production was very low indeed, since his ion current amounted to less than one micro-ampere. (A mass spectrograph in which one micro-ampere of normal uranium ions passes through the separating fields to the collectors will collect about one microgram of U-235 per 16-hour day.) The great need of samples of enriched U-235 for nuclear study was recognized early by Lawrence, who decided to see what could be done with the help of the 37-inch (cyclotron) magnet at Berkeley. The initial stages of this work were assisted by a grant from the Research Corporation of New York, which was later repaid. Beginning January 1, 1942, the entire support came from the OSRD through the S-1 Committee. Later, as in other parts of the uranium project, the contracts were taken over by the Manhattan District.
11.5. At Berkeley, after some weeks of planning, the 37-inch cyclotron was dismantled on November 24, 1941, and its magnet was used to produce the magnetic field required in what came to be called a "calutron" (a name representing a contraction of "California University cyclotron"). An ion source consisting of an electron beam traversing the vapour of a uranium salt was set up corresponding to the ion source shown in the drawing in Chap. IX, p. 164. Ions were then accelerated to the slit S2 through which they passed into the separating region where the magnetic field bent their paths into semicircles terminating at the collector data. By December 1, 1941, molecular ion beams from the residual gas were obtained, and shortly thereafter the beam consisting of singly charged uranium ions (U+) was brought up to an appreciable strength. It was found that a considerable proportion of the ions leaving the source were U+ ions. For the purpose of testing the collection of separated samples, a collector with two pockets was installed, the two pockets being separated by a distance appropriate to the mass numbers 235 and 238. Two nail collection runs using U+ beams of low strength were made in December, but subsequent analyses of the samples showed only a small separation factor. By the middle of January 1942, a run had been made with a reasonable beam strength and an aggregate flow or through-put of appreciable amount which odd a much improved separation factor. By early February 1942, beams of much greater strength were obtained, and Lawrence reported that good separation factors were obtainable with such beams. By early March 1942, the ion current had been raised still further. These results tended to bear out Lawrence's hopes that space charge could be neutralized by ionisation of the residual gas in the magnet chamber.
INITIATION OF A LARGE PROGRAM
11.6. By this time it was clear that the calutron was potentially able to effect much larger scale separations than had ever before been approached by an electromagnetic method. It was evidently desirable to explore the whole field of electromagnetic separation. With this end in view, Lawrence mobilized his group at the Radiation Laboratory of the University of California at Berkeley and began to call in others to help. Among those initially at Berkeley were D. Kicks, P. C. Aebersold, W. M. Brobeck, F. A. Jenkins, K. R. MacKenzie, W. B. Reynolds, D. H. Sloan, F. Oppenheimer, J. G. Backs, B. Peters, A. C. Helmholz, T. Finkelstein, and W. E. Perkins, Jr. Lawrence called back some of his former students, including R. L. Thornton, J. R. Richardson, and others. Among those working at Berkeley for various periods were L. P. Smith from Cornell, E. U. Condon and J. Slepian from Westinghouse, and I. Langmuir and K. H. Kingdom from General Electric. During this early period J. R. Oppenheimer was still at Berkeley and contributed some important ideas. In the fall of 1943 the group was further strengthened by the arrival of a number of English physicists under the leadership of M. L. Oliphant of the University of Birmingham.
11.7. Initially a large number of different methods were considered and many exploratory experiments were performed. The main effort, however, soon became directed towards the development of the calutron, the objective being a high separation factor and a large current in the positive ion beam.
IMMEDIATE OBJECTIVES
11.8. Of the three apparent limitations listed in the first paragraph - difficulty of producing ions, limited fraction of ions actually used, and space charge effects - only the last had yielded to the preliminary attack. Apparently space charge in the neighborhood of the positive ion beam could be nullified to a very great extent. There remained as the immediate objectives a more productive ion source and more complete utilization of the ions. 11.9. The factors that control the effectiveness of an ion source are many. Both the design of the source proper and the method of drawing ions from it are involved. The problems to be solved cannot be formulated simply and must be attacked by methods that are largely empirical. Even if security restrictions permitted an exposition of the innumerable forms of ion source and accelerating system that were tried, such exposition would be too technical to present here.
11.10. Turning to the problem of effecting more complete utilization of the ions, we must consider in some detail the principle of operation of the calutron. The calutron depends on the fact that singly charged ions moving in a uniform magnetic field perpendicular to their direction of ion are bent into circular paths of radius proportional to their momenta. Considering now just a single isotope, it is apparent that the ions passing through the two slits (and thus passing into the large evacuated region in which the magnetic field is present) do not initially follow a single direction, but have many initial directions lying within a small angle, whose size depends on the width of the slits.
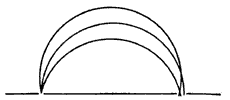
Fortunately, however, since all the ions of the isotope in question follow curved paths of the sam diameter, ions starting out in slightly different directions tend to meet again - or almost meet again - after completing a semicircle. It is, of course, at this position of reconvergence that the collector is placed. Naturally, the ions of another isotope (for example, ions of mass 238 instead of 235) behave similarly, except that they follow circles of slightly different diameter. Samples of the two isotopes lawyer caught in collectors at the two different positions of reconvergence. Now the utilization of a greater fraction of the ions originally produced may be accomplished readily enough by widening the two slits referred to. But to widen the slits to any great extent without sacrificing sharpness of focus at the reconvergence positions is not easy. Indeed it can be accomplished only by use of carefully proportioned space variations in the magnetic field strength. Fortunately, such variations were worked out successfully.
11.11. Another problem, not so immediate but nevertheless recognized as important to any production plant, was that of more efficient use of the magnetic field. Since large electromagnets are expensive both to build and to operate, it was natural to consider using the same magnetic field for several ion beams. The experimental realisation of such an economical scheme became a major task of the laboratory.
THE GIANT MAGNET
11.12. Although the scale of separation reached by March 1942 was much greater than anything that had previously been done with an electromagnetic mass separator, it was still very far from that required to produce amounts of material that would be of military significance. The problems that have been outlined not only had to be solved, but they had to be solved on a grand scale. The 37-inch cyclotron magnet that had been used was still capable of furnishing useful information, but larger equipment was desirable. Fortunately a very much larger magnet, intended for a giant cyclotron, had been under construction at Berkeley This magnet, with a pole diameter of 184 inches and a pole gap of 72 inches, was to be the largest in existence. Work on it had been interrupted because of the war, but it was already sufficiently advanced so that it could be finished within a few months if adequate priorities were granted. Aside from the magnet itself, the associated building, laboratories, shops, etc., were almost ideal for the development of the calutron. Needless to say, work was resumed on the giant magnet and by the end of May 1942 it was ready for use. *
11.13. The first experiments using the 37-inch magnet have been described in a previous paragraph. Later developments proceeded principally along these two lines: construction and installation of a properly engineered separation unit for the 37-inch magnet, and design and construction of experimental separation units to go into the big magnet.
11.14. Besides the gradual increase in ion beam strength and separation factor that resulted from a series of developments in the ion source and in the accelerating system, the hoped-for improvement in utilization of ions was achieved during the summer of 1942, using the giant magnet. Further, it was possible to maintain more than one ion beam in the same magnetic separating region. Experiments on this latter problem did run into some difficulties, however, and it appeared that there might be limitations on the number of sources and receivers that could be put in a single unit as well as on the current that could be used in each beam without spoiling the separation.
11.15. It was evident that many separator units would be needed to get an amount of production of military significance. Therefore, consideration was given to various systems of combining groups of units in economical arrangements. A scheme was worked out which was later used in the production plants and which has proved satisfactory.
ADVANTAGEOUS OF THE ELECTROMAGNETIC SYSTEM
11.16. In September 1942, both the gaseous diffusion and the centrifugal methods of uranium isotope separation had been under intensive study - and for a longer period than in the case of the electromagnetic method. Both of these methods - gaseous diffusion and centrifuge - looked feasible for large-scale production of U-235, but both would require hundreds of stages to achieve large-scale separation. Neither had actually produced any appreciable amounts of separated U-235. No large-scale plant for plutonium production was under way, and the self sustaining chain reaction which was to produce plutonium had not yet been proved attainable. But in the case of the electromagnetic method, after the successful separation of milligram amounts, there was no question as to the scientific feasibility. If one unit could separate 10 mg a day, 100,000,000 units could separate one ton a day. The questions were of cost and time. Each unit was to be a complicated electromagnetic device requiring high vacuum, high voltages, and intense magnetic fields: and a great deal of research and development work would be required before complete, large-scale, units could be constructed. Many skilled operators would probably be needed. Altogether, at that time it looked very expensive, but it also looked certain and relatively quick. Moreover, the smallness of the units had the advantage that development could continue, modifications could be made in the course of construction or, within limits, after construction, and capacity could always be expanded by building new units.
POLICY QUESTION
11.17. On the basis of rather incomplete scientific and engineering information on all the methods and on the basis of equally dubious cost estimates, decisions had to be made on three issues: (1) whether to build an electromagnetic plant; (2) how big such a plant should be; (3) at what point of development the design should be frozen.
APPROVAL OF PLANT CONSTRUCTION
11.18. On the strength of the results reported on experiments at Berkeley in the summer of 1942, the S-1 Executive Committee at a meeting at Berkeley on September 13-14, 1942, recommended that commitments be made by the Army for an electromagnetic separation plant to be built at the Tennessee Valley site (Clinton Engineer Works). It was recommended that it should be agreed that commitments for this plant might be cancelled on the basis of later information. It was recommended that a pilot plant should be erected at the Tennessee Valley site as soon as possible. (However, this recommendation was subsequently withdrawn and such a pilot plant was never built.) The construction of a production plant was authorized by General Groves on November 5, 1942, with the understanding that the design for the first units was to be frozen immediately.
ORGANIZATION FOR PLANNING AND CONSTRUCTION
11.19. In describing the production of plutonium, we discussed the division of responsibility between the Metallurgical Project and the duPont Company. The electromagnetic separation plant was planned and built under a somewhat different scheme of organization. The responsibility was divided between six major groups. The Radiation Laboratory at the University of California was responsible for research and development; the Westinghouse Electric and Manufacturing Company for making the mechanical parts, i.e., sources, receivers, pumps, tanks, etc..; the General Electric Company for the electrical equipment and controls the Allis-Chalmers Company for the magnets; the Stone and Webster Engineering Company for the construction and assembly; and the Tennessee Eastman Company for operation. All five industrial concerns kept groups of their engineers at Berkeley so that a system of frequent informal conference and cross- checking was achieved. Thus the major part of the planning was one cooperatively in a single group, even though the details might be left to the home offices of the various companies.
THE BASIS OF THE TECHNICAL DECISIONS
11.20. Strangely enough, although the theory of the self sustaining chain-reacting pile is already well worked out, the theory of gaseous discharge, after fifty years of intensive study, is still inadequate for the prediction of the exact behavior of the ions in a calutron. The amount of U-235 collected per day, and the purity of the material collected, are affected by many factors, including: (1) the width, spacing, and shape of the collector, (2) the pressure in the magnet space, (3) the strength and uniformity of the magnetic field, (4) the shape and spacing of the defining slits and accelerating system, (5) the accelerating voltage (6) the size and shape of the slit in the arc source from which the ions come, (7) the current in the arc, (8) the position of the arc within the arc chamber, (9) the pressure of vapor in the arc chamber, (10) the chemical nature of the vapor. Evidently there was not time for a systematic study of all possible combinations of variables. The development had to be largely intuitive. A variety of conditions had to be studied and a number of partial interpretations had to be made. Then the accumulated experience of the group, the "feel" of the problem, had to be translated into specific plans and recommendations.
TECHNICAL DECISIONS REQUIRED
11.21. (a) The Number of Stages. As in all methods, a compromise must be made between yield and separation factor. In the electromagnetic system, the separation factor is much higher than in other systems so that the number of stages required is small. There was a possibility that a single stage might be sufficient. Early studies indicated that attempts to push the separation factor so high as to make single-stage operation feasible cut the yield to an impracticably small figure.
11.22. (b) Specifications. The information and experience that had been acquired on the variables such as those mentioned above had to be translated into decisions on the following principal points before design could actually begin: (1) the size of a unit as determined by the radius of curvature of the ion path the length of the source slit, and the arrangement of source and receivers; (2) the maximum intensity of magnetic field required; (3) whether or not to use large divergence of ion beams: (4) the number of ion sources and receivers per unit; (5) whether the source should be at high potential or at ground potential; (6) the number of accelerating electrodes and the maximum potentials to be applied to them; (7) the power requirements tar arcs, accelerating voltages, pumps, etc.; (8) pumping requirement, (9) number of units per pole gap; (10) number of units per building.
EXPERIMENTAL UNITS AT BERKELEY
11.23. Most of the design features for the first plant had to be frozen in the fall of 1942 on the basis of results obtained with runs made using the giant magnet at Berkeley. The plant design, however, called for units of a somewhat different type. While there was no reason to suppose that these changes would introduce any difference in performance, it was obviously desirable to build a prototype unit at Berkeley. The construction of this unit was approved at about the same time that the first plant units were ordered so that experience with it had no influence on fundamental design, but it was finished and operating by April 1943, that is, six months before the first plant unit. Consequently, it was invaluable for testing and training purposes. Later, a third magnet was built in the big magnet building at Berkeley. All told, there have been six separator units available simultaneously for experimental or pilot plant purposes at Berkeley. Much auxiliary work has also been done outside the complete units.
ISOTRON SEPARATOR
11.24. A we have already said, H. D. Smyth of Princeton became interested in electromagnetic methods of separation in the late summer and fall of 1941. He was particularly interested in devising some method of using an extended ion source and beam instead of one limited essentially to one dimension by a system as in the calutron mass separator. A method of actually achieving separation using an extended ion source was suggested by R. R. Wilson of Princeton. The device which resulted from Wilson's ideas was given the deliberately meaningless name "isotron."
11.25. The isotron is an electromagnetic mass separator using extended source of ions, in contrast to the slit sources used in ordinary mass spectrographs. The ions from the extended source are first accelerated by a constant, high-intensity, electric field and are then further accelerated by a low-intensity electric field varying at radio frequency and in "saw tooth" manner. The effect of the constant electric field is to project a strong beam of ions down a tube with uniform kinetic energy and therefore with velocities inversely proportional to the square root of the masses of ions. The varying electric field, on the other hand, introduces small, periodic variations in ion velocity, and has the effect of causing the ions to "bunch" at a certain distance down the tube. (This same principle is used in the klystron high- frequency oscillator, where the electrons are "bunched" or "velocity-modulated.") The bunches of ions of different mass travel with different velocities and therefore become separated. At the position (actually on area perpendicular to the beam where this occurs, an analyzer applies a transverse focussing electric field with a radio frequency component synchronized with the arrival of the bunches. The synchronization is such that the varying component of the transverse field strength is zero when the U-235 ion bunches come through and a maximum when the U-238 ion bunches come through. The U-235 beams are focussed on a collector, but the U-238 bunches are deflected Thus the separation is accomplished.
11.26. This scheme was described at the December 18, 1941 meeting of the Uranium Committee and immediately thereafter was discussed more fully with Lawrence, who paid a visit to Princeton. The promise of the method seemed sufficient to justify experimental work, which was begun immediately under an OSRD contract and continued until February 1943. Since the idea involved was a novel one, there were two outstanding issues: (1) whether the method would work at all; (2) whether it could be developed for large-scale production promptly enough to compete with the more orthodox methods already under development.
11.27. An experimental isotron was constructed and put into operation by the end of January 1942. Preliminary experiments at that time indicated that the isotopes of lithium could be separated by the method. The first successful collection of partially separated uranium isotopes was made in the spring
11.28. Unfortunately, progress during the summer and fall of 1942 was not as rapid as had been hoped. Consequently, it was decided to close down the Princeton project in order to permit sending the personnel to the site where the atomic-bomb laboratory was about to get under way. Before the group left Princeton a small experimental isotron collected several samples of partly separated uranium. Thus, the method worked; but its large-scale applicability was not fully investigated.
THE MAGNETRON AND THE IONIC CENTRIFUGE
11.29. In December 1941, when the whole subject of isotope separation was under discussion at Berkeley, the magnetron was suggested as a possible mass separator. In the meantime, Smyth of Princeton had been in contact with L. P. Smith of Cornell and had discovered that Smith and his students had done a considerable amount of work - and with evidence of success - on the separation of the isotopes of lithium by just such a method. This was reported to Lawrence in Washington at one of the December, 1941, meetings of the Uranium Committee. Lawrence immediately got in touch with Smith, with the result that Smith worked on the method at Berkeley from February 1942 to June 1942. J. Slepian of the Westinghouse Research Laboratory in East Pittsburgh came to Berkeley in the winter of 1941-1942 at Lawrence's invitation and became interested in a modification of the magnetron which he called an ionic centrifuge. Slepian stayed at Berkeley most of the time until the fall of 1942, after which he returned to East Pittsburgh where he continued the work.
11.30. No separation of uranium was actually attempted in the magnetron. Experiments with lithium with low ion currents showed some separation, but no consistent results were obtained with high ion currents. In the case of the ionic centrifuge, uranium samples have been collected showing appreciable separation, but the results have not been clear-cut or consistent.
THE SITUATION AS OF EARLY 1943
11.31. With the virtual elimination of the isotron and the ionic centrifuge from the development program, the calutron separator became the only electromagnetic method worked on intensively. Construction of initial units of a plant had been authorized and designs had been frozen for such units, but the whole electromagnetic program had been in existence for only a little more than a year and it was obvious that available designs were based on shrewd guesses rather than on adequate research. A similar situation might have occurred with the chain reacting pile if unlimited amounts of uranium and graphite had been available before the theory had been worked out or before the nuclear constants had been well determined. Fortunately the nature of the two projects was very different, making it a less speculative venture to build an electromagnetic plant unit hastily than would have been the case for the pile. Further research and development could proceed advantageously even while initial units of the plant were being built and operated.
CONSTRUCTION AND OPERATION; MARCH 1943 TO JUNE 1945
COMPARISON WITH DIFFUSION AND PLUTONIUM PLANTS
11.32. The preceding chapters show that the end of 1942 was a time of decision throughout the uranium project. For it was at that time that a self-sustaining chain reaction was first produced, that construction was authorized for the Hanford plutonium plant, the diffusion plant at Clinton, and the electromagnetic plant at Clinton. The diffusion plant was more flexible than the plutonium plant, since the diffusion plant could be broken down into sections and stages, built in whole or in part, to produce varying amounts of U-235 of varying degrees of enrichment. The electromagnetic plant was even more flexible, since each separator unit was practically independent of the other units. The separation process consisted of loading a charge into a unit, running the unit for a while, then stopping it and removing the product. To be sure, the units were built in groups, but most of the controls were separate for each unit. This feature made it possible to build the plant in steps and to start operating the first part even before the second was begun. It was also possible to change the design of subsequent units as construction proceeded; within limits it was possible even to replace obsolescent units in the early groups with new improved units.
NATURE AND ORGANIZATION OF DEVELOPMENT WORK
11.33. Construction of the first series of electromagnetic units at Clinton began in March of 1943 and this part of the plant was ready for operation in November 1943. The group at Berkeley continued to improve the ion sources, the receivers, and the auxiliary equipment, aiming always at greater ion currents. In fact, Berkeley reports describe no less than seventy-one different types of source and one hundred and fifteen different types of receiver, all of which reached the design stage and most of which were constructed and tested. As soon as the value of a given design change was proved, every effort was made to incorporate it in the designs of new units.
11.34. Such developments as these required constant interchange of information among laboratory, engineering, construction, and operating groups. Fortunately the liaison was excellent. The companies stationed representatives at Berkeley, and members of the research group at Berkeley paid frequent and prolonged visits to the plant at Clinton. In fact, some of the research men were transferred to the payroll of the Tennessee Eastman Company operating the plant at Clinton, and a group of over one hundred physicists and research engineers still kept on the Berkeley payroll were assigned to Clinton. Particularly in the early stages of operation the Berkeley men stationed at Clinton were invaluable as "trouble shooters" and in instructing operators. A section of the plant continued to be maintained as a pilot unit for testing modified equipment and revised operating procedures, and was run jointly by the Berkeley group and by Tennessee Eastman. In addition to the British group under Oliphant already mentioned, there was a British group of chemists at Clinton under J. W. Baxter.
CHEMICAL PROBLEMS
11.35. Originally, the uranium salts used as sources of vapor for the ion-producing arcs had not been investigated with any very great thoroughness at Berkeley, but as the process developed, a good deal of work was done on these salts, and a search was made for a uranium compound that would be better than that originally used. Some valuable studies were also made on methods of producing the compound chosen.
11.36. By far the most important chemical problem was the recovery of the processed uranium compounds from the separation units. This recovery problem had two phases. In units of the first stage it was essential to recover the separated uranium from the receivers with maximum efficiency; whereas recovery of the scattered unseparated uranium from other parts of the unit was less important. But if higher stage units are used even the starting material contains a high concentration of U-235, and it is essential to recover all the material in the unit at the end of each run, i.e., material remaining in the ion source and material deposited on the accelerating electrodes, on the walls of the magnet chamber, and on the receiver walls.
THE THERMAL DIFFUSION PLANT
11.37. For nearly a year the electromagnetic plant was the only one in operation. Therefore the urge to increase its production rate was tremendous. It was realized that any method of enriching - even slightly enriching - the material to be fed into the plant would increase the production rate appreciably. For example, an electromagnetic unit that could produce a gram a day of 40 per cent pure U-235 from natural uranium could produce two grams a day of 80 per cent U-235 if the concentration of U-235 in the feed material was twice the natural concentration (1.4 per cent instead of 0.7 per cent).
11.38. We have already referred to the work done by P. H. Abelson of the Naval Research Laboratory on the separation of the uranium isotopes by thermal diffusion in a liquid compound of uranium. By the spring of 1943 Abelson had set up a pilot plant that accomplished appreciable separation of a considerable quantity of uranium compound. It was therefore proposed that a large-scale thermal diffusion plant should be constructed. Such a plant would be cheaper than any of the other large-scale plants, and it could be built more quickly. Its principal drawback was its enormous consumption of steam, which made it appear impracticable for the whole job of separation.
11.39. Not only was a pilot plant already in operation at the Naval Research Laboratory, but a second, somewhat larger plant was under construction at the Philadelphia Navy Yard. Through the cooperation of the Navy both the services of Abelson and the plans for a large-scale plant were made available to the Manhattan District. It was decided to erect the large-scale thermal diffusion plant at Clinton (using steam from the power plant constructed for the gaseous diffusion plant) and to use the thermal-diffusion-plant product as feed material for the electromagnetic plant.
11.40. This new thermal diffusion plant was erected in amazingly short time during the late summer of 1944. In spite of some disappointments, operation of this plant has succeeded in its purpose of considerably increasing the production rate of the electromagnetic plant. It has also stimulated work on the uranium recovery problem. The future of this plant is uncertain. Operation of the gaseous-diffusion plant makes it difficult to get enough steam to operate the thermal diffusion plant, but also furnishes another user for its product.
MISCELLANEOUS PROBLEMS
11.41. Although the scientific and technical problems which confronted the Berkeley groups were probably not as varied or numerous as the problems encountered at Chicago and Columbia, they were nevertheless numerous. Thus many problems arose in the designing of the electric power and control circuits, magnetic fields, insulators, vacuum pumps, tanks, collectors, and sources. Many equipment items had to be designed from scratch and the mass-produced under high priority.
PRESENT STATUS
11.42. The electromagnetic separation plant was in large-scale operation during the winter of 1944-1945, and produced U-235 of sufficient purity for use in atomic bombs. Its operating efficiency is being continually improved. Research work is continuing although on a reduced scale
SUMMARY
11.43. In the early days of the uranium project, electromagnetic methods of isotope separation were rejected primarily because of the expected effects of space-charge. In the fall of 1941 the question was reopened; experiments at Berkeley showed that space-charge effects could be largely overcome. Consequently a large-scale program for the development of electromagnetic methods was undertaken.
11.44. Of the various types of electromagnetic methods proposed, the calutron (developed at Berkeley) received principal attention. Two other novel methods were studied, one at Berkeley and one at Princeton. The calutron mass separator consists of an ion source from which a beam of uranium ions is drawn by an electric field, an accelerating system in which the ions are accelerated to high velocities, a magnetic field in which the ions travel in semicircles of radia, depending on ion mass, and a receiving system. The principal problems of this method involved the ion source, accelerating system, divergence of the ion beam, space charge, and utilization of the magnetic field. The chief advantages of the calutron were large separation factor, small hold-up, short start-up time, and flexibility of operation. By the fall of 1942 sufficient progress had been made to justify authorization of plant construction, and a year later the first plant units were ready for trial at the Clinton Engineer Works in Tennessee
11.45. Research and development work on the calutron were carried out principally at the Radiation Laboratory of the University of California, under the direction of Lawrence. Westinghouse, General Electric, and Allis Chalmers constructed a majority of the parts; Stone and Webster built the plant, and Tennessee Eastman operated it.
11.46. Since the calutron separation method was one of batch operations in a large number of largely independent units, it was possible to introduce important improvements even after plant operation had begun.
11.47. In the summer of 1944 a thermal-diffusion separation plant was built at the Clinton Engineer Works to furnish enriched feed material for the electromagnetic plant and thereby increase the production rate of this latter plant. The design of the thermal diffusion plant was based on the results of research carried out at the Naval Research Laboratory and on the pilot plant built by the Navy Department at the Philadelphia Navy Yard.
11.48. Although research work on the calutron was started later than on the centrifuge and diffusion systems, the calutron plant was the first to produce large amounts of the separated isotopes of uranium.